Spin orbit torque (SOT) represents a groundbreaking advancement in spintronics. By leveraging quantum forces, scientists can control magnetic states using electric currents. This control opens doors to faster, more efficient memory devices and logic circuits. To harness SOT’s full potential, we must understand its microscopic origins. These origins lie in the intricate interplay between an electron’s spin and its orbital motion. Let’s explore the fascinating world of quantum mechanics to uncover the secrets behind spin orbit torque.
Spin Orbit Interaction and Its Role in Spin Orbit Torque
Spin orbit interaction (SOI) forms the backbone of SOT, a pivotal concept in spintronics. Understanding SOI is essential for grasping the microscopic origins of SOT. Here, we explore the basics of SOI and how it drives the phenomenon of spin orbit torque.
Understanding Spin Orbit Interaction
Spin orbit interaction arises from the interplay between an electron’s spin and its motion around an atomic nucleus. In simple terms, when an electron orbits a nucleus, it experiences an electric field due to the nucleus’s positive charge. According to special theory of relativity, in the electron’s frame of reference, this electric field transforms into a magnetic field. This magnetic field interacts with the electron’s magnetic moment, which originates from its spin, resulting in spin orbit coupling.
Mathematically, SOI can be described using the Hamiltonian:
$H_{SOI}$=λL⋅S
Here, λ represents the spin orbit coupling constant, L denotes the orbital angular momentum, and S stands for the spin angular momentum. This equation illustrates that the energy of an electron depends on the relative orientation of its spin and orbital angular momentum.
Role of Heavy Metals and Spin Current Generation
Heavy metals, such as platinum, gold, and tantalum, exhibit strong spin orbit coupling due to their large atomic numbers. When an electric current passes through these materials, electrons scatter off impurities and lattice imperfections. This scattering process generates a spin current perpendicular to the charge current. The spin Hall effect (SHE) primarily drives this generation of spin current. In SHE, electrons with opposite spins deflect in opposite directions, creating a transverse spin current.
Rashba Effect and Topological Insulators
The Rashba effect also plays a significant role in enhancing SOI. In materials lacking inversion symmetry, such as at surfaces or interfaces, the SOI splits the spin bands. This splitting results in spin-polarized currents. Topological insulators, which feature conducting surfaces and insulating bulk, provide another platform for strong SOI. Their surface states exhibit robust spin-momentum locking, meaning the electron’s spin orientation is directly tied to its momentum. This property is crucial for efficient spin current generation and manipulation.
Microscopic Mechanisms Leading to Spin Orbit Torque
To comprehend the microscopic mechanisms of SOT, consider the behavior of individual electrons in a heavy metal. As electrons move through the material, their spins align with the effective magnetic field generated by SOI. This alignment creates a spin accumulation at the interface with an adjacent ferromagnetic layer. The accumulated spins exert a torque on the magnetic moments in the ferromagnet, causing them to precess or switch.
Additionally, the spin current generated via SHE or the Rashba effect transfers angular momentum to the ferromagnetic layer. This transfer of angular momentum results in spin orbit torque, which can manipulate the magnetic state of the ferromagnet.
Damping-Like and Field-Like Spin Orbit Torque: Microscopic Origins and Differences
SOT encompasses two primary components: damping-like SOT and field-like SOT. Each component arises from distinct microscopic mechanisms and impacts magnetic dynamics differently. Understanding these differences is crucial for optimizing spintronic devices.
Damping-Like Spin Orbit Torque
Damping-like SOT primarily results from the spin Hall effect (SHE) in heavy metals. When an electric current passes through a heavy metal, such as platinum or tantalum, it generates a transverse spin current due to SHE. Electrons with opposite spins deflect in opposite directions, creating a net spin accumulation at the interface with an adjacent ferromagnetic layer.
This spin accumulation exerts a torque on the magnetic moments in the ferromagnetic layer. The torque aligns with the damping term in the Landau-Lifshitz-Gilbert (LLG) equation, which describes the dynamics of the magnetization (M):
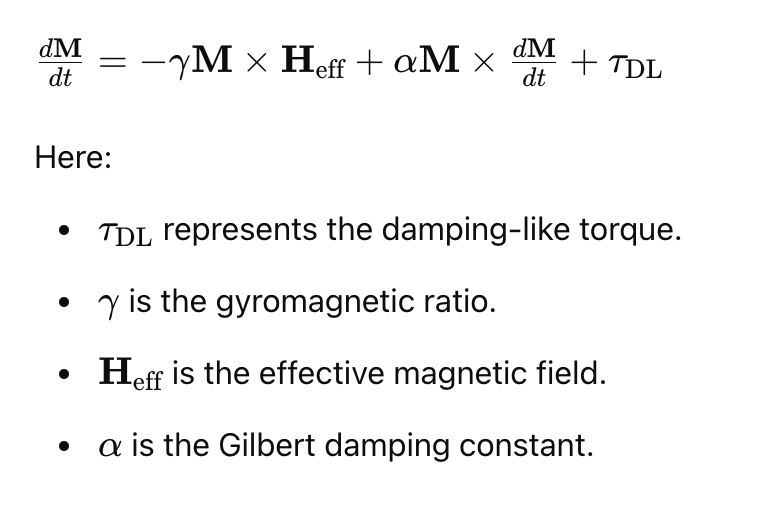
The damping-like torque $τ_{DL}$ aligns with M×(M×S), where S is the spin accumulation vector. This torque efficiently drives magnetization switching and domain wall motion, making it highly effective for memory applications like magnetic random-access memory (MRAM).
Field-Like Spin Orbit Torque
Field-like SOT, on the other hand, arises from the Rashba-Edelstein effect at interfaces with broken inversion symmetry. When an electric current flows through such an interface, it generates a non-equilibrium spin density due to the Rashba effect. This spin density acts like an effective magnetic field, known as the Rashba field, which exerts a torque on the magnetization.
The field-like torque $τ_{FL}$ adds a term to the LLG equation:

The $τ_{FL}$ aligns with M×S. This torque mimics the effect of an external magnetic field applied perpendicular to the current direction. While less efficient for switching applications compared to damping-like torque, field-like torque can assist in precessional dynamics and enhance the overall control of magnetization.
Microscopic Origins: Key Differences
The microscopic origins of damping-like and field-like SOTs differ fundamentally:
- Damping-Like SOT:
- Originates from the spin Hall effect in bulk heavy metals.
- Requires strong spin orbit coupling and significant spin Hall angle.
- Spin accumulation occurs at the heavy metal/ferromagnet interface.
- Primarily influences magnetization through angular momentum transfer.
- Field-Like SOT:
- Stems from the Rashba-Edelstein effect at interfaces with broken inversion symmetry.
- Requires strong spin orbit coupling and significant Rashba parameter.
- Non-equilibrium spin density forms at the interface.
- Mimics an effective magnetic field acting on the magnetization.
Experimental Validation
Experimental evidence supports the theoretical understanding of SOI and its role in SOT. Techniques like spin-polarized scanning tunneling microscopy (SP-STM) reveal spin accumulation at interfaces. Measurements of the spin Hall angle, which indicates the efficiency of spin current generation, align well with theoretical predictions. These experiments validate the significance of SOI in the generation and control of spin currents
The title image visually captures electrons spiraling like a cyclone, illustrating their dynamic orbital motion around a nucleus. This motion highlights the relativistic effects experienced by electrons due to their high velocity. According to relativity, moving electrons perceive the electric field from the nucleus as an effective magnetic field. This transformation arises from the interplay between the electron’s spin and orbital motion, leading to spin orbit interaction. This interaction, in turn, creates the torque that drives phenomena like spin orbit torque. The cyclonic pattern represents the complex behavior of electrons under these relativistic conditions, emphasizing how spin and orbital motion combine to produce significant effects in spintronics
Title Image Description
Endnote
Thank you for taking the time to read “Harnessing Quantum Forces: The Microscopic Origins of Spin Orbit Torque.” Your interest in exploring the fascinating world of spintronics means a lot. I would greatly appreciate any feedback you may have, as it helps me improve and provide more valuable content. If you enjoyed this article and want to stay updated on future posts, please consider subscribing to our Website. Your support is crucial in helping us grow and continue delivering insightful articles. Thank you once again for your time and engagement!