Magnetic skyrmions are nanoscale vortices of spins that promise to revolutionize data storage and processing with their unique, energy-efficient properties.
Magnetic skyrmions represent a groundbreaking discovery in materials science. These tiny structures, resembling swirling whirlpools of spins, promise to revolutionize data storage and processing technologies. Let’s explore what makes magnetic skyrmions so remarkable and their potential impact on future innovations.
A magnetic skyrmion is a stable, localized configuration of magnetic spins. In simple terms, it looks like a swirling vortex of spins. At the center, the spins point upwards. As you move outward, the spins gradually twist and point downwards. This twisting motion forms a stable and compact magnetic structure.
Why Skyrmions Are Important?
Magnetic skyrmions are crucial due to their unique properties, which offer significant advantages for technology and materials science. Their importance stems from their stability, small size, and low energy manipulation.
Stability and Size
Skyrmions exhibit remarkable stability due to their specific spin configuration. At the microscopic level, skyrmions form a vortex-like structure where spins twist from an upward direction at the core to a downward direction at the edges. This configuration minimizes energy and provides stability.
Mathematically, the stability of skyrmions can be described using the topological charge Q. This charge quantifies the winding number of the spin configuration and is given by:

Here, n represents the unit vector of the spin orientation, and the integral is taken over a surface that encompasses the skyrmion. The topological charge Q remains constant, ensuring the skyrmion’s stability.
Low-Energy Manipulation
Skyrmions can be manipulated with very low energy, which is highly beneficial for data storage and processing. The energy required to move a skyrmion is significantly lower compared to traditional magnetic domains. This low energy requirement arises because skyrmions are stabilized by their topological structure rather than by large exchange interactions.
The energy of a skyrmion $E_{sk}$ can be approximated by considering the exchange interaction and the Dzyaloshinskii-Moriya interaction (DMI). The exchange energy density $E_{ex}$ and DMI energy density $E_{DMI}$ contribute to the total energy:

Here, A is the exchange stiffness, D is the Dzyaloshinskii-Moriya interaction constant, and n represents the spin orientation.
High-Density Data Storage
Skyrmions’ small size makes them ideal for high-density data storage. Unlike conventional magnetic domains, which require larger areas, skyrmions occupy a much smaller footprint. This property enables the packing of more information into a smaller space, leading to higher data storage densities.
The size of a skyrmion R depends on the material properties and can be expressed as:
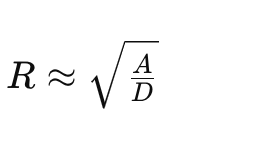
where A is the exchange stiffness and D is the Dzyaloshinskii-Moriya interaction constant. Smaller skyrmions translate to higher density data storage.
Efficient Data Processing
Skyrmions can move and interact with minimal energy consumption. This efficiency results from their stable, low-energy configuration. In spintronic devices, skyrmions could potentially lead to faster and more energy-efficient data processing.
The dynamics of skyrmions are described by the Thiele equation, which models the motion of skyrmions under an applied current:
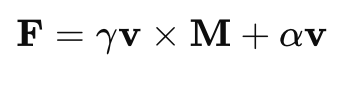
where F is the force acting on the skyrmion, γ is the gyromagnetic ratio, v is the velocity of the skyrmion, M is the magnetization, and α is the damping parameter. The low-energy movement enables efficient manipulation of skyrmions.
The Formation of Skyrmions
Skyrmions form through a delicate interplay of magnetic forces and material properties. These structures appear in specific magnetic materials under certain conditions. Understanding how skyrmions develop involves examining the roles of magnetic interactions, spin-orbit coupling, material properties, material thickness, magnetic anisotropy and external factors.
Magnetic Interactions
The first step in skyrmion formation involves magnetic interactions within the material. Magnetic materials have regions where spins align in a particular way due to exchange interactions. In some cases, these interactions can lead to the formation of skyrmions. They create a stable, swirling configuration of spins.
Spin-Orbit Coupling
Spin-orbit coupling plays a crucial role in stabilizing skyrmions. This phenomenon arises from the interaction between the electron’s spin and its orbital motion around the nucleus. Strong spin-orbit coupling in certain materials helps maintain the skyrmion’s structure, allowing it to persist even when other forces might disrupt it.
Material Properties
The type of material significantly influences skyrmion formation. Thin magnetic films and specific compounds often exhibit the right conditions for skyrmions to form. For instance, materials with a chiral magnetic interaction are particularly favorable. These materials help stabilize the skyrmion structure and support its formation.
Material Thickness
The thickness of the magnetic material also impacts skyrmion formation. In thin magnetic films, the reduced dimensionality often enhances the conditions for skyrmion stability. Thin films can exhibit unique magnetic properties and interactions that support the formation and maintenance of skyrmions.
Magnetic Anisotropy
Magnetic anisotropy refers to the directional dependence of a material’s magnetic properties. In materials with strong magnetic anisotropy, the spins prefer to align along specific directions. This preference can stabilize skyrmions by providing a preferred orientation for the spin configuration, helping to maintain the skyrmion structure.
External Factors
Material properties are often influenced by external factors such as temperature and magnetic fields. For instance, materials with specific temperature ranges exhibit the right conditions for skyrmions to form. Similarly, applying a magnetic field can help stabilize skyrmions in materials that otherwise might not support their formation.
Applications of Skyrmions
Magnetic skyrmions hold immense potential in various technological fields due to their unique properties. These tiny, stable spin structures offer advantages like low-energy manipulation, high-density data storage, and fast processing. Here’s a detailed look at how skyrmions can transform multiple applications.
1. Data Storage Devices
One of the most promising applications of skyrmions lies in data storage. Skyrmions are nanoscale magnetic structures, much smaller than conventional magnetic domains. This allows for the development of high-density storage devices. More data can fit into a smaller area, significantly increasing storage capacity.
The stability of skyrmions, ensured by their topological protection, means they can retain information reliably. Researchers are exploring how to write, move, and delete skyrmions using electric currents with minimal energy, making storage devices more energy-efficient. Future hard drives and solid-state devices could use skyrmions to achieve greater storage capacities while consuming less power.
2. Spintronic Devices
Skyrmions could revolutionize spintronics, a field that uses the electron’s spin rather than its charge for information processing. Skyrmions can move in a material with low electric currents, reducing power consumption. This makes them ideal for spintronic devices, which aim to combine storage and logic functions within a single architecture.
For example, skyrmion-based racetrack memory uses skyrmions as information carriers. In these devices, skyrmions move along a nanowire when a small current is applied, representing bits of information. This technology could lead to faster, more efficient memory devices with longer lifespans than traditional flash memory.
3. Neuromorphic Computing
Skyrmions have potential applications in neuromorphic computing, which aims to mimic the human brain’s neural networks for efficient data processing. The ability of skyrmions to form stable, controllable structures and interact in complex ways makes them suitable for creating artificial synapses and neurons.
Researchers are investigating skyrmion-based devices to replicate synaptic functions. In these devices, skyrmion movement and interaction can mimic the adaptive learning processes of biological synapses. This approach could pave the way for low-power, highly efficient artificial intelligence systems that better replicate human cognitive functions.
4. Quantum Computing
Skyrmions might play a role in quantum computing. Their topological properties make them candidates for stable qubits, the building blocks of quantum computers. Skyrmions exhibit quantum mechanical effects at the nanoscale, and their stability could help maintain quantum coherence.
Using skyrmions as qubits could lead to quantum computers that are less prone to errors caused by decoherence. This stability, coupled with their ability to be controlled precisely, makes them attractive for developing quantum bits that are robust against environmental noise.
5. Sensors and Actuators
Skyrmions also show promise in sensor and actuator technologies. Their sensitivity to external magnetic fields and electric currents allows for the development of highly responsive magnetic sensors. Skyrmion-based sensors could detect minute changes in magnetic fields, offering high sensitivity and precision.
In actuator applications, skyrmions could enable precise control over magnetic forces and movements. This could be useful in microscale robotics and other technologies requiring fine magnetic control.
6. Magnetic Logic Devices
Skyrmions offer potential for magnetic logic devices that perform computing tasks using magnetic states rather than electrical charges. These devices could operate with less energy and produce less heat, making them ideal for future computing architectures.
By using skyrmions in magnetic logic gates, researchers could develop circuits that perform logical operations more efficiently than traditional semiconductor-based devices. This approach could lead to new kinds of computing devices that combine processing and storage, reducing latency and improving overall efficiency.
Conclusion
Skyrmions are important due to their stable, low-energy spin configurations and their potential for high-density data storage and efficient processing. Their microscopic properties, including the topological charge and energy considerations, make them ideal for next-generation technologies. As research advances, skyrmions may become integral to developing more efficient and compact devices.
Share Your Thoughts!
Thank you for taking the time to explore the fascinating world of magnetic skyrmions with us! Your feedback is invaluable, so please share your thoughts and questions in the comments section below. Consider to visit the folder spintronics for more articles from same domain and subscribe to our blog for more insights and updates on cutting-edge science and technology. Stay tuned, and we look forward to engaging with you in the next article!